Laser Induced Breakdown Spectroscopy (LIBS)
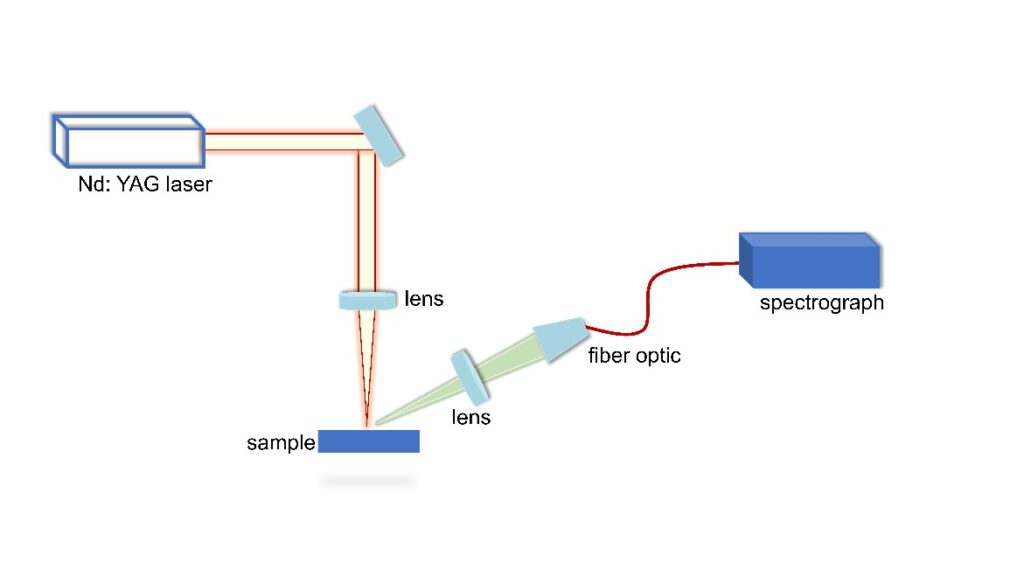
A typical LIBS setup is basically consisted of a pulsed laser, some optics to focus the laser beam on the sample to create a spark, i.e. a microplasma, some lenses or fiber to transfer the light emitted from the plasma to a spectrograph to be spectrally analyzed and some detector to record the spectrum.
Our laboratory bears all the necessary equipment for performing LIBS quantitative and qualitative analyses, while there is versatility and technical knowledge for performing other laser-based techniques, too (e.g. Laser-Induced Fluorescence spectroscopy). A variety of laser sources is available, with laser pulse durations ranging from fs to ns time scales and wavelengths ranging from UV to IR. Moreover, there is a plethora of instruments for spectral acquisition, from VUV up to IR wavelength ranges.
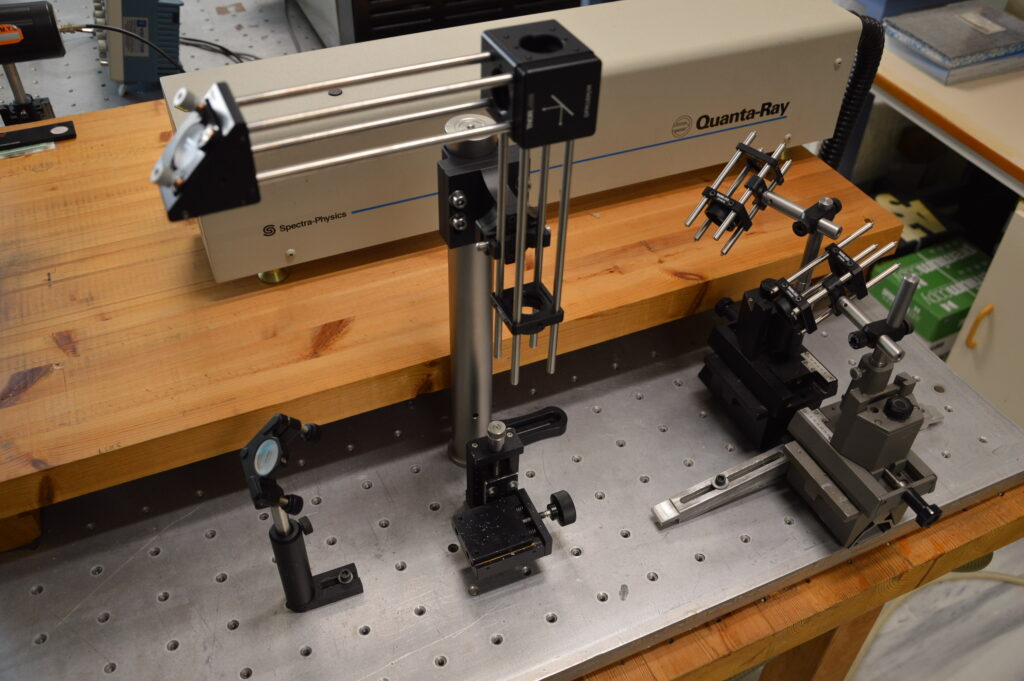
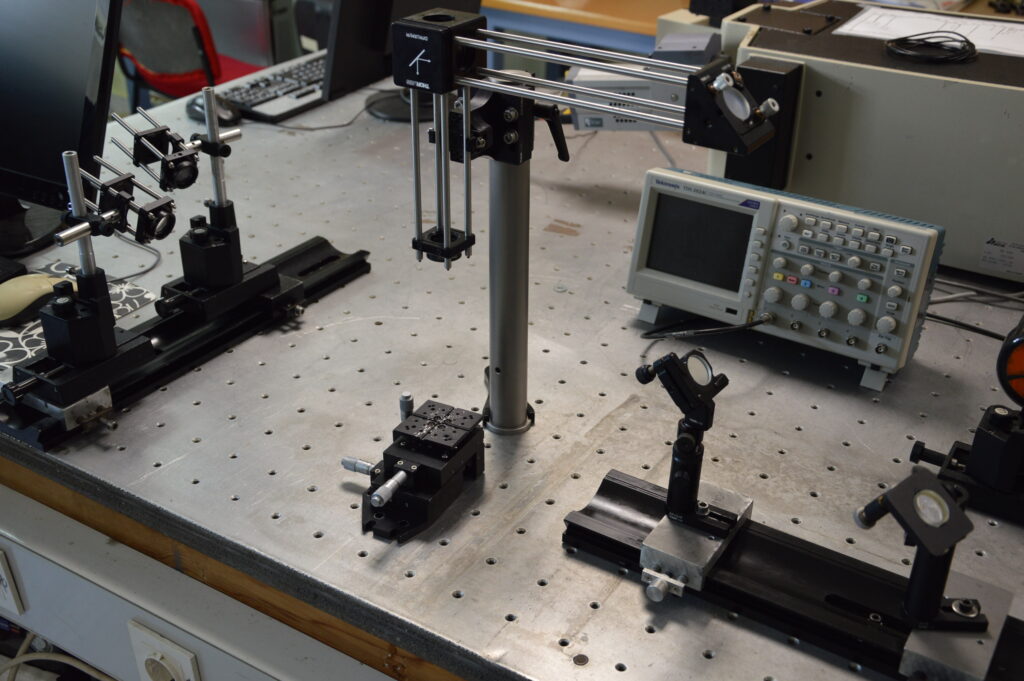
Z-scan Technique
The Z-scan is a laser based technique allowing the simultaneous determination of both the magnitude and the sign of the NLO absorption and refraction of a sample from a single measurement. According to this technique the normalized transmittance of a sample translated along the propagation direction (e.g. the z-axis) of a focused Gaussian laser beam is measured by two different experimental configurations, the so-called “open-aperture” (OA) and “closed- aperture” (CA) Z-scans. In the case of closed-aperture transmission measurement, the transmitted through the sample laser beam is measured, after it has passed through a narrow pinhole placed in the far field. An OA Z-scan can exhibit a transmission minimum or maximum, indicating reverse saturable absorption (RSA) or saturable absorption (SA) behaviour respectively. The CA Z-scan recording exhibits a pre-focal transmission valley followed by a post-focal transmission peak or a pre-focal transmission pick followed by a post-focal transmission valley. The former behaviour suggests self-focusing and the latter self-defocusing behaviour respectively.
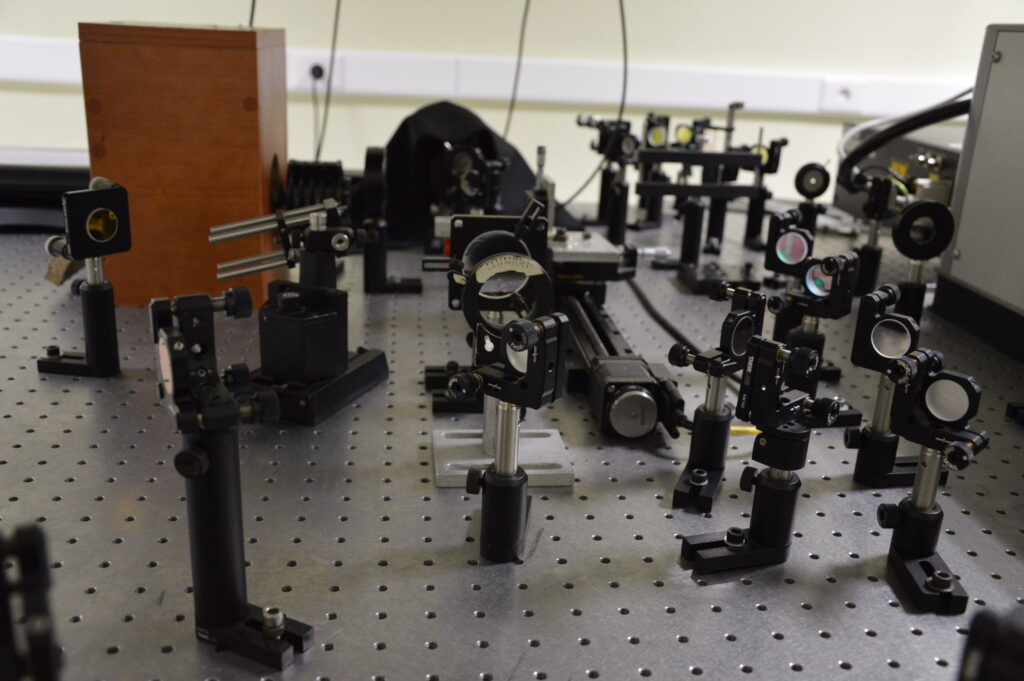
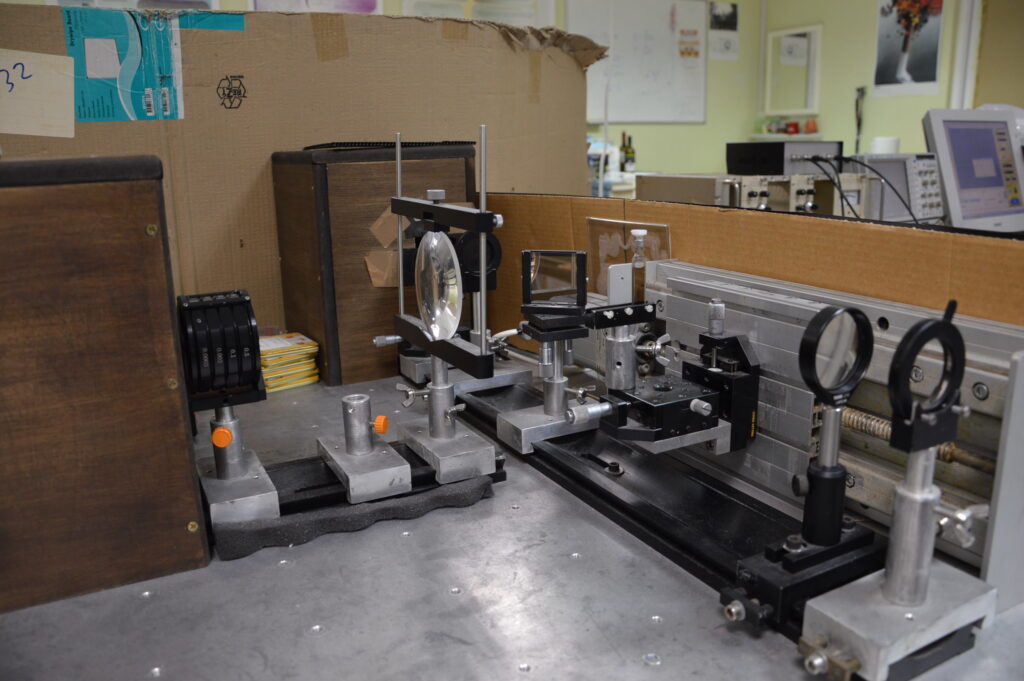
Optical Kerr Effect Technique (OKE)
OKE is a pump-probe technique, often used to study the magnitude of the third order NLO response of materials and its temporal behavior. In general, OKE permits the determination of χ(3) by comparison with a reference material, when absorption is low or negligible. According to this technique, a laser beam is split in a strong pump and a weak probe beams, with the polarization of the former set at 45o with respect to the probe by means of a Glan Taylor polarizer. A computer-controlled translation stage allows to vary the time delay between the pump and probe beams, which are then combined into the sample by means of a 20 cm focal length quartz lens. The strong pump beam induces a refractive index anisotropy in the nonlinear medium, while the weak probe beam “reads” the induced anisotropy, after passing through a Glan Taylor polarizer positioned perpendicularly to its initial polarization direction. The “leaking” probe beam measured by a PMT is the OKE signal.
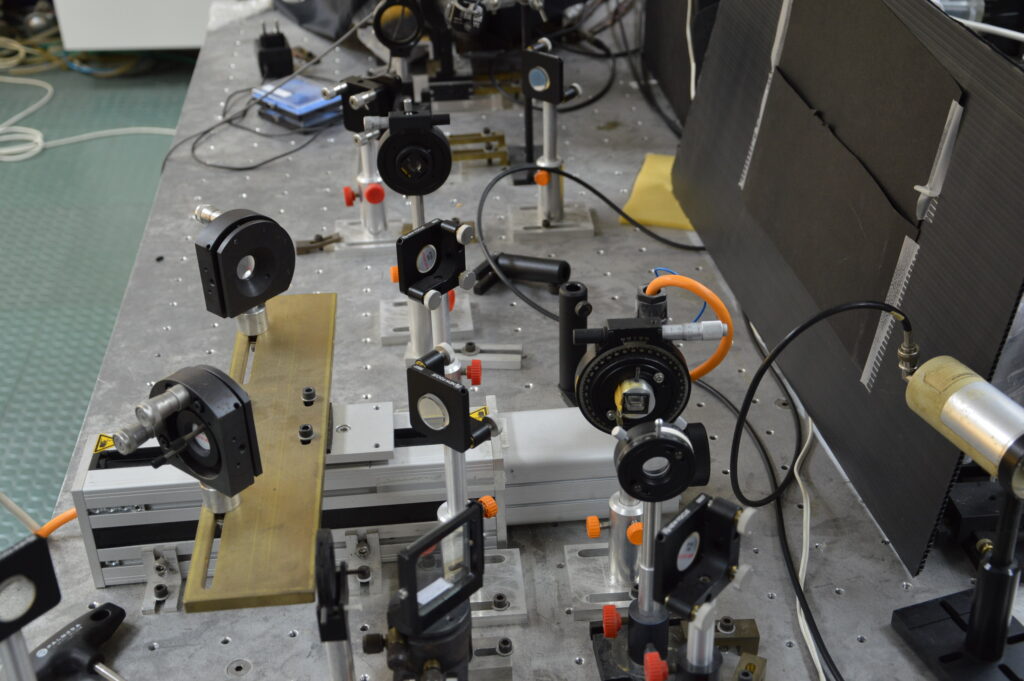
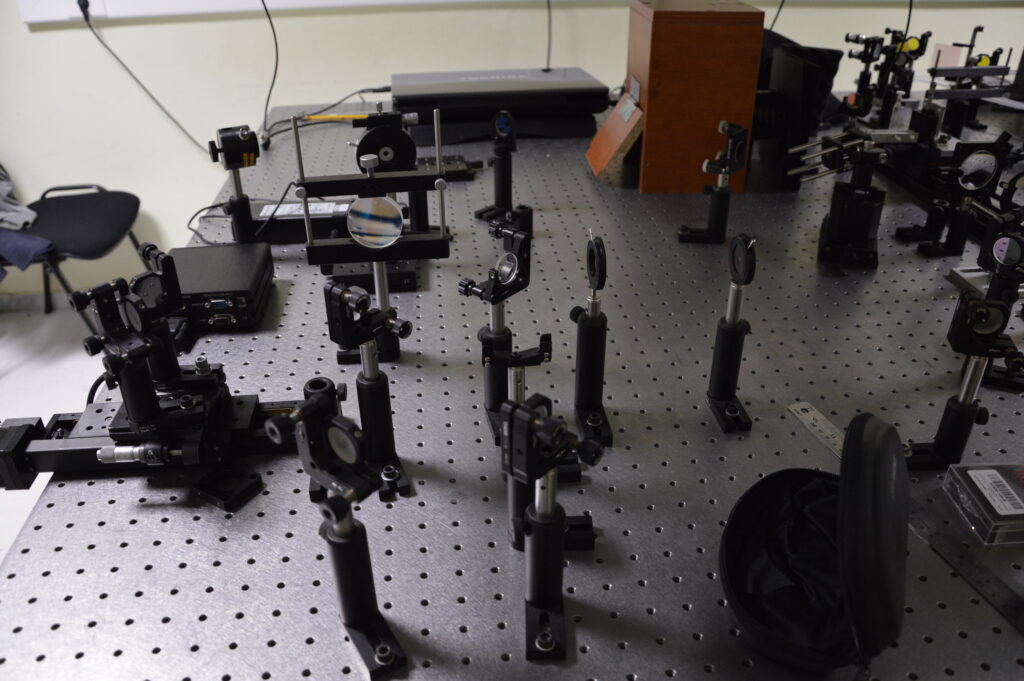
Thermal lensing Technique combined with Z-scan
Most of the studies investigating the NLO properties of graphene and graphene derivatives under fs laser excitation are performed using high repetition rate lasers ranging from kilohertz (kHz) to megahertz (MHz), while studies using low repetition rate lasers are rather scarce. Nevertheless, in the former case, a relatively strong manifestation of thermal cumulative effects can be enabled, mainly in the case where the measurements are conducted by means of the Z-scan technique, overwhelming the otherwise quite weaker mechanism of electronic origin contributions on the NLO response. A simple experimental method capable of distinguishing the electronic contributions from the thermo-optical effects is the conventional single beam Z-scan technique combined with a thermal lensing measurement technique, which was firstly proposed by Falconieri and Salvetti. This experimental technique is used of our group to confirm the existence of thermal cumulative effects in graphenes under high repetition rate pulses excitation.
According to this technique, a mechanical chopper placed just after the output of a Ti:Sapphire laser system oscillator (delivering 800 nm, 200 fs, 80 MHz pulses) is used to produce a train of pulses, their number being defined by the laser repetition rate and the frequency of the chopper wheel. Thus, resulting to the exposure of a sample to a variable period laser irradiation conditions. The transmitted laser light is detected by a photodetector (PMT) placed along the CA Z-scan arm of the Z-scan experimental configuration, measuring the variations of the sample’s transmission after the laser beam has passed through an iris positioned at the far-field. The PMT’s output is integrated by a boxcar integrator. The gate of the boxcar is positioned at different time delays from the chopper opening rise time and onwards. In this way, the temporal evolution of the CA Z-scan, and therefore the temporal evolution of the thermal effects due to the sample different irradiation conditions, is possible to be probed. In fact, the measurements were conducted by recording the sample transmittance at the far-field by means of the CA Z-scan as a function of the time delay, during a cycle where the laser beam is not interrupted by the chopper blade.
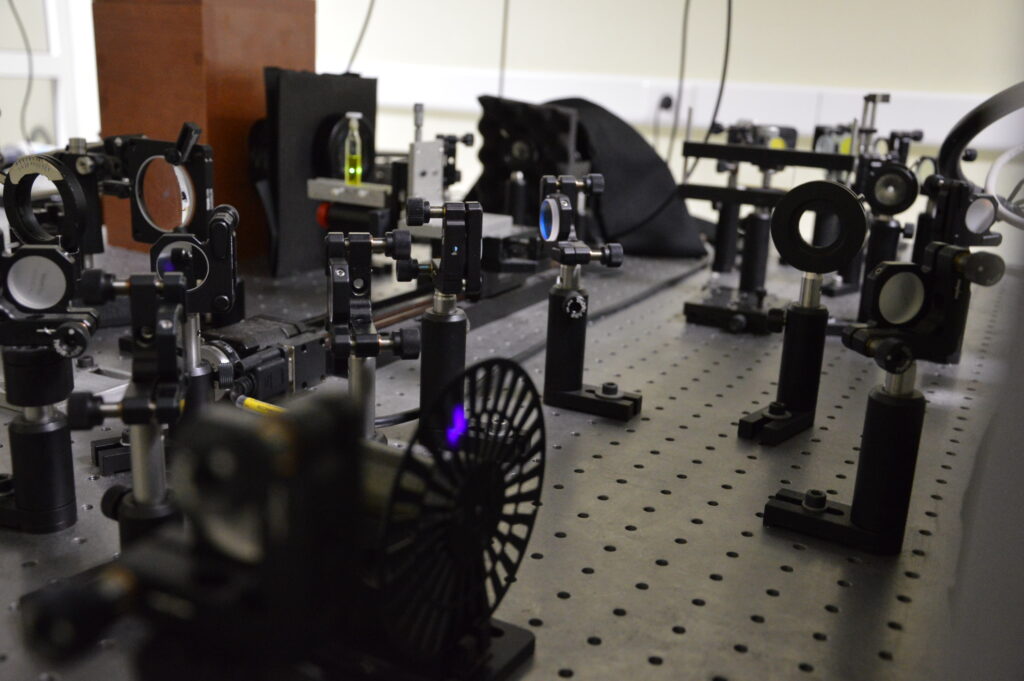